Sustainable batteries: an ongoing challenge to circular thinking
The recycling of batteries is an ongoing challenge worth our active engagement. Understanding circular economy principles can bring us closer to zero-emission vehicles
By Marja Rinne (Aalto University, Finland).
In our everyday lives, we tend to think of recycling as something that happens when we diligently sort our waste into different fractions and toss them into their respective bins without much thought to what happens next. While continuing to do that is extremely important - after all, nothing would get recycled if it was not collected - it is worthwhile considering what a complex chain of processing steps your waste undergoes once someone empties the bins.
The reason why sorting is important is that it simplifies the separation of different materials into different process streams: metal cans require different treatment steps from paper and plastic. However, many pieces of equipment we use daily contain many components and materials, which further complicates the issue. The separation process is even more critical when parts of the recycled goods are at risk of catching fire or causing environmental damage if managed improperly. An example of such hazardous waste is lithium-ion batteries, which are powering the shift towards electric road transport.
The role of recycling in the sustainability of electrification cannot be understated.
Electric vehicle (EV) batteries are massive, weighing anything from 100 kg to 500 kg, and bind considerable amounts of natural resources within them. Without a recycling infrastructure, we would need to replace the landfilled lithium, cobalt, graphite, copper, and other raw materials by mining virgin materials, further contributing to environmental pollution. Furthermore, batteries are flammable and contain toxic metals and chemicals, which are at risk of being released into the environment over time if they are not dealt with correctly.
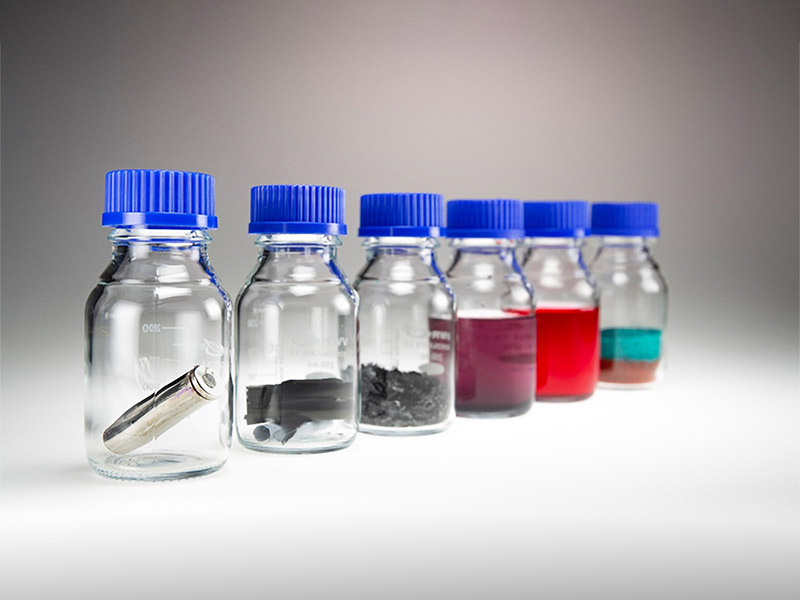
Figure 2: Battery cells go through complex process routes before the metals are recovered as pure metals. From left to right: intact battery cell, separated electrode materials, crushed batteries (black mass), cobalt-rich leaching solution, purified cobalt solution, nickel sulphate (green layer) on top of pure cobalt sulphate (red layer). Source: Valeria Azovskaya, 2019
Although we can agree that effective recycling is critical, it is by no means simple. Like so many of our everyday products, we are still not designing lithium-ion batteries with recycling in mind. An old lead-acid battery comprises approximately 60% lead, while the rest is mainly sulfuric acid. But a typical lithium-ion cell only contains 2-3% lithium and about 20% of other elements, such as cobalt, nickel, and manganese.
Current recycling processes aim to recover mainly valuable cobalt, copper, and nickel from the batteries, leaving most of the other materials lost in the waste streams.
The recovery of lithium, for example, has been non-existent to date, but interest in its reclamation has grown due to concerns over resource scarcity. Nevertheless, many other component materials, including graphite, phosphate, manganese, and aluminium, have received less attention due to their lower values.
A combination of different unit processes is needed to maximize the effective recovery of valuable materials. The typical recycling processes combine mechanical, pyrometallurgical, and hydrometallurgical steps at various stages. In pyrometallurgical processes, we use high temperatures are used to divide the metals into molten metal alloy and disposable slag. In hydrometallurgical processes, the metals are leached from shredded battery waste (or “black mass”) using acid solutions. Both options have their advantages and disadvantages.
Although we used to rely mainly on pyrometallurgical techniques, the so-called “black mass” routes are gaining momentum due to the pressure to recover more valuables from the waste. The new EU regulatory framework for batteries (EU Battery Regulation) includes mandatory minimum levels of recycled material content for cobalt, nickel, lead, and lithium, and minimum material recovery targets for lithium, cobalt, nickel, copper, and lead. The reclamation of lithium is more manageable if we opt for leaching solutions. This is one of the reasons why the Aalto University School of Engineering engages in the development and environmental life cycle evaluation—along with the Fundació Institut de Recerca en Energia de Catalunya (IREC)—of state-of-the-art hydrometallurgical processes that are based on black mass within the HELIOS project. It is easy to imagine that these processes are complex, due to the high number of elements that need to be separated and purified.
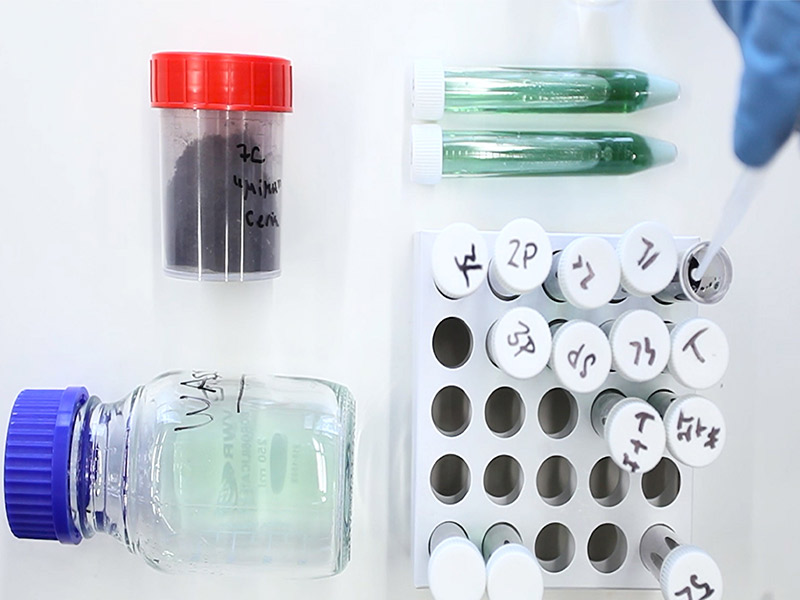
Nonetheless, with highly chemically heterogeneous waste inputs, the related process chain becomes highly complicated: after all, if the battery mix includes a combination of lithium- nickel-manganese-cobalt-oxide (NMC) and lithium-iron-phosphate (LFP) cells, recovering all the valuable materials would mean to separate and purify copper, aluminium, lithium, nickel, manganese, cobalt, iron, phosphorus, graphite, plastics, and the electrolyte. A whole lot of very different components!
By estimation, approximately 10 to 40% of the cell mass can be recovered with current technologies. Near future black mass processes, which reclaim lithium and ensure higher recovery efficiencies are obtained for nickel, cobalt, and copper, are expected to reach 15 to 60% cell mass recovery, depending on cathode chemistry. Nickel and cobalt-rich lithium-cobalt-oxide (LCO), nickel-cobalt-aluminium-oxide (NCA), and nickel-manganese-cobalt-oxide (NMC) materials are simpler to recycle, while lower value LFP and lithium-manganese-oxide (LMO) are more challenging. No viable recovery routes currently exist for any of the potential anode materials. Future batteries, such as lithium-sulphur and lithium-air, are expected to contain even fewer high-value materials, which is why entirely new recycling solutions are likely to be needed soon.
Even though nickel and cobalt-based battery chemistries have their advantages in terms of recycling, they do have other drawbacks. For instance, the value chain of cobalt has become well known for the egregious human rights violations occurring in the Democratic Republic of Congo, which holds by far the world’s largest cobalt deposits. And the problems raised by the nickel value chain should also not be ignored. The materials in LMO and LFP chemistries are better in terms of social concerns, but their lower energy density means that, for an equal capacity, more battery mass is needed. This also means that a larger quantity of component materials is necessary, which is a complex umbrella problem to disentangle.
The circular economy is not a goal that can be reached by first designing products and then finding ways to recycle them once they are out in the market: product designers need to consider circular thinking needs since the start of product development. Circular economy needs and principles must be further pointed out during the entire product development process, to ensure no recycling aspect is forgotten or under-estimated. Circular thinking must be applied to electric batteries, as optimized recycling routes support zero-emission goals by decreasing the need for intensive virgin material production.
Such a concept is at the heart of the EU-funded HELIOS project, which aims to integrate circularity and life cycle assessment in the optimal design of EV battery packs. In the project, Aalto University collaborates with different partners including Bozankaya, Technical University of Sophia, Danish Technological Institute and European Copper Institute to improve the design and materials selection of battery pack components. This eco(circular) design approach aims to help ensure that the dismantling processes at the end of life allow for a more straightforward recovery of recyclable materials through mechanical and hydrometallurgical processing. Furthermore, this innovative and holistic approach, embodied by the HELIOS project, is paramount for the successful attainment of the goals set out by the EU Green Deal and the truly zero-emission vehicles needed for a better future.
If you’re curious to learn more on the HELIOS project and cleaner batteries,keep an eye on our news!